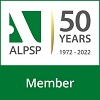
Assessment of the Solar Energy Potential over South America Estimated by CMIP6 Models in the Present and Future Climate
DOI:
https://doi.org/10.30564/jees.v6i2.6425Abstract
Developing the renewable energy matrix of South America (SA) is fundamental for sustainable socioeconomic growth and mitigating climate change's adverse effects. Thus, this study estimates changes in SA's solar irradiance and solar power potential using data from eight global climate models (GCMs) belonging to the Coupled Model Intercomparison Project—Phase 6 (CMIP6). Applying statistical downscaling and bias correction with the Quantile Delta Mapping (QDM) technique, we evaluate projected changes in the Concentrated Solar Power (CSP) and Photovoltaic Power (PVP) outputs under different future climate scenarios (SSP2-4.5 and SSP5-8.5). Historical simulations (1995–2014) are validated using ERA5 reanalysis and CLARA-A3 satellite observations. The QDM method reduces the models' systematic biases, decreasing the ensemble's errors by 50% across SA throughout the year. Regarding future decades (2020–2099), the CMIP6 ensemble shows spatial and seasonal variability in solar generation. For CSP, estimates suggest that regions traditionally favorable to solar energy generation (such as the Brazilian Northeast and portions of Chile) will maintain their suitable conditions during the 21st century, projecting a potential 1–6% increase (particularly under the SSP5-8.5 scenario in southern Chile and most of Brazil). Concerning PVP generation, the CMIP6 ensemble projects a rise of 1–4% (mainly under the SSP5-8.5 scenario in the Amazonia, Midwest, and Southeast Brazilian sectors). Moreover, trend analyses projected individually by the CMIP6 GCMs converge on an increasing PVP, mainly in Brazil's Amazonia and Midwest regions. In contrast, for South Brazil, approximately 84% of the projections show a negative trend (or no trend), evidencing unfavorable or uncertain conditions for solar generation development in the region. Despite the data and processes' inherent limitations, this study yields a first analysis of statistically downscaled projections from CMIP6 for solar power generation in South America, providing valuable information for energy sector decision-makers.
Keywords:
Solar power; CMIP6; Climate change; Statistical downscaling; South AmericaReferences
[1] Climate Change 2023: Synthesis Report. IPCC: Geneva, Switzerland. pp. 1–34. DOI: https://doi.org/10.59327/IPCC/AR6-9789291691647.001
[2] Reboita, M.S., Ambrizzi, T., 2022. Climate system in a nutshell: an overview for understanding climate change. International Journal of Development Research. 12, 53365-53378. Available from: https://www.journalijdr.com/sites/default/files/issue-pdf/23731.pdf
[3] Climate Change: Global Temperature [Internet] [cited 2024 Apr 3]. Available from: https://www.energy.gov/sites/default/files/2024-02/093.%20Rebecca%20Lindsey%20and%20Luann%20Dahlman%2C%20NOAA%2C%20Climate%20Change_%20Global%20Temperature.pdf
[4] Icaza, D., Borge-Diez, D., Galindo, S.P., 2022. Analysis and proposal of energy planning and renewable energy plans in South America: Case study of Ecuador. Renewable Energy. 182, 314–342. DOI: https://doi.org/10.1016/j.renene.2021.09.126
[5] Arango-Aramburo, S., Ríos-Ocampo, J.P., Larsen, E.R., 2020. Examining the decreasing share of renewable energy and growing thermal capacity: The case of South America. Renewable and Sustainable Energy Review. 119, 109648. DOI: https://doi.org/10.1016/j.rser.2019.109648
[6] Deng, Q., Alvarado, R., Toledo, E., Caraguay, L., 2020. Greenhouse gas emissions, non-renewable energy consumption, and output in South America: the role of the productive structure. Environmental Science and Pollution Research. 27, 14477–14491. DOI: https://doi.org/10.1007/s11356-020-07693-9
[7] A Race to the Top Latin America 2023: Wind and Solar Utility-Scale Buildout Gains Speed in Brazil, Chile and Colombia, while Mexico Falls Behind [Internet] [cited 2024 Apr 5]. Available from: https://globalenergymonitor.org/report/a-race-to-the-top-latin-america/
[8] Brazil Holds the Key to Latin America's Solar Potential [Internet] [cited 2024 Apr 5]. Available from: https://www.reuters.com/markets/commodities/brazil-holds-key-latin-americas-solar-potential-2024-02-29/#:~:text=PV%20PLANS,of%20Latin%20America's%20solar%20generation
[9] Exame, E.S.G., 2024. Solar Energy Reaches 40 GW of Installed Capacity in Brazil. Retrieved from Exame ESG [Internet] [cited 2024 Apr 5]. Available from: https://exame.com/esg/energia-solar-alcanca-40-gw-de-capacidade-instalada-no-brasil/ (In Portuguese)
[10] Patt, A., Pfenninger, S., Lilliestam, J., 2013. Vulnerability of solar energy infrastructure and output to climate change. Climatic Change. 121, 93–102. DOI: https://doi.org/10.1007/s10584-013-0887-0
[11] Solar Energy [Internet] [cited 2024 Apr 5]. Available from: https://www.irena.org/Energy-Transition/Technology/Solar-energy
[12] Letcher, T.M., 2022. Comprehensive renewable energy, 2nd ed. Elsevier: Amsterdam, Netherlands. pp. 796.
[13] Solar Energy Becomes the Third Largest Source in Brazil [Internet] [cited 2024 Apr 5]. Available from: https://www.gov.br/en/government-of-brazil/latest-news/solar-energy-becomes-the-third-largest-source-in-brazil
[14] Crook, J.A., Jones, L.A., Forster, P.M., et al., 2011. Climate change impacts on future photovoltaic and concentrated solar power energy output. Energy & Environmental Science. 4, 3101. DOI: https://doi.org/10.1039/c1ee01495a
[15] Jerez, S., Tobin, I., Vautard, R., et al, 2015. The impact of climate change on photovoltaic power generation in Europe. Nature Communications. 6, 10014. DOI: https://doi.org/10.1038/ncomms10014
[16] Wild, M., Folini, D., Henschel, F., Fischer, N., Müller, B., 2015. Projections of long-term changes in solar radiation based on CMIP5 climate models and their influence on energy yields of photovoltaic systems. Solar Energy. 116, 12–24. DOI: https://doi.org/10.1016/j.solener.2015.03.039
[17] Müller, J., Folini, D., Wild, M., et al., 2019. CMIP-5 models project photovoltaics are a no-regrets investment in Europe irrespective of climate change. Energy. 171, 135–148. DOI: https://doi.org/10.1016/j.energy.2018.12.139
[18] Zou, L., Wang, L., Li, J., et al., 2019. Global surface solar radiation and photovoltaic power from Coupled Model Intercomparison Project Phase 5 climate models. Journal of Cleaner Production. 224, 304–324. DOI: https://doi.org/10.1016/j.jclepro.2019.03.268
[19] Feron, S., Cordero, R.R., Damiani, A., et al., 2021. Climate change extremes and photovoltaic power output. Nature Sustainability. 4, 270–276. DOI: https://doi.org/10.1038/s41893-020-00643-w
[20] Gernaat, D.E.H.J., de Boer, H.S., Daioglou, V., Yalew, S.G., Müller, C., van Vuuren, D.P., 2021. Climate change impacts on renewable energy supply. Nature Climate Change. 11, 119-125. DOI: https://doi.org/10.1038/s41558-020-00949-9
[21] Sawadogo, W., Reboita, M.S., Faye, A., et al, 2021. Current and future potential of solar and wind energy over Africa using the RegCM4 CORDEX‑CORE ensemble. Climate Dynamics. 57, 1647–1672. DOI: https://doi.org/10.1007/s00382-020-05377-1
[22] Dutta, R., Chanda, K., Maity, R., 2022. Future of solar energy potential in a changing climate across the world: A CMIP6 multimodel ensemble analysis. Renewable Energy. 188, 819–829. DOI: https://doi.org/10.1016/j.renene.2022.02.023
[23] Ndiaye, A., Moussa, M.S., Dione, C., et al., 2023. Projected changes in solar PV and wind energy potential over West Africa: an analysis of CORDEX-CORE simulations. Energies. 15, 9602. DOI: https://doi.org/10.3390/en15249602
[24] Ha, S., Zhou, Z., Im, E.-S., et al., 2023. Comparative assessment of future solar power potential based on CMIP5 and CMIP6 multimodel ensembles. Renewable Energy. 206, 324–335. DOI: https://doi.org/10.1016/j.renene.2023.02.039
[25] Barbosa, L.S.N.S., Bogdanov, D., Vainikka, P., et al., 2017. Hydro, wind and solar power as a base for a 100% renewable energy supply for South and Central America. PLoS ONE. 12, e0173820. DOI: https://doi.org/10.1371/journal.pone.0173820
[26] Gil, G.M.V., Cunha, R.B.A., Di Santo, S.G., et al, 2020. Photovoltaic energy in South America: Current state and grid regulation for large-scale and distributed photovoltaic systems. Renewable Energy. 162, 1307–1320. DOI: https://doi.org/10.1016/j.renene.2020.08.022
[27] Narvaez, G., Giraldo, L.F., Bressan, M., 2022. The impact of climate change on photovoltaic power potential in Southwestern Colombia. Heliyon. 8, e11122. DOI: https://doi.org/10.1016/j.heliyon.2022.e11122.
[28] Lopez, G., Aghahosseini, A., Bogdanov, D., et al, 2021. Pathway to a fully sustainable energy system for Bolivia across power, heat, and transport sectors by 2050. Journal of Cleaner Production. 293, 126195. DOI: https://doi.org/10.1016/j.jclepro.2021.126195
[29] Osorio-Aravena, J.C., Aghahosseini, A., Bogdanov, D., et al, 2021. The impact of renewable energy and sector coupling on the pathway towards a sustainable energy system in Chile. Renewable and Sustainable Energy Reviews. 151, 111557. DOI: https://doi.org/10.1016/j.rser.2021.111557
[30] de Jong, P., Barreto, T.B., Tanajura, C.A.S., et al, 2019. Estimating the impact of climate change on wind and solar energy in Brazil using a South American regional climate model. Renewable Energy. 141, 390–401. DOI: https://doi.org/10.1016/j.renene.2019.03.086
[31] Costa, R.S., da Costa, G.L., de Lima, F.J.L., et al, 2020. Impacts of climate change on the availability of solar energy resources. Revista Brasileira de Energia. 26, 39–50. (In Portuguese). DOI: https://doi.org/10.47168/rbe.v26i4.579
[32] Zuluaga, C.F., Avila-Diaz, A., Justino, F.B., et al, 2022. The climate change perspective of photovoltaic power potential in Brazil. Renewable Energy. 193, 1019–1031. DOI: https://doi.org/10.1016/j.renene.2022.05.029
[33] Ambrizzi, T., Reboita, M.S., da Rocha, R.P., et al., 2019. The state of the art and fundamental aspects of regional climate modeling in South America. Annals of the New York Academy of Sciences. 1436, 98–120. DOI: https://doi.org/10.1111/nyas.13932
[34] Mutz, S.G., Scherrer, S., Muceniece, I., et al., 2021. Twenty-frst century regional temperature response in Chile based on empirical-statistical downscaling. Climate Dynamics. 56, 2881–2894. DOI: https://doi.org/10.1007/s00382-020-05620-9
[35] Ballarin, A.S., Sone, J.S., Gesualdo, G.C., et al, 2023. CLIMBra—Climate change dataset for Brazil. Scientific Data. 10, 47. DOI: https://doi.org/10.1038/s41597-023-01956-z
[36] Cannon, A.J., Sobie, S.R., Murdock, T.Q., 2015. Bias correction of GCM precipitation by Quantile Mapping: How well do methods preserve changes in quantiles and extremes? Journal of Climate. 28, 6938–6959. DOI: https://doi.org/10.1175/JCLI-D-14-00754.1
[37] Ferreira, G.W.S., Reboita, M.S., 2022. A new look into the South America precipitation regimes: Observation and forecast. Atmosphere. 13, 873. DOI: https://doi.org/10.3390/atmos13060873
[38] Rupp, D.E., Abatzoglou, J.T., Hegewisch, K.C., et al., 2013. Evaluation of CMIP5 20th century climate simulations for the Pacific Northwest USA. Journal of Geophysical Research: Atmosphere. 118, 10884–10906. DOI: https://doi.org/10.1002/jgrd.50843
[39] Ferreira, G.W.S., Reboita, M.S., Ribeiro, J.G.M., et al., 2023. Assessment of precipitation and hydrological droughts in South America through statistically downscaled CMIP6 projections. Climate. 11, 166. DOI: https://doi.org/10.3390/cli11080166
[40] Ferreira, G.W.S., Reboita, M.S., Ribeiro, J.G.M., et al., 2024. Assessment of the wind power density over South America simulated by CMIP6 models in the present and future climate. Climate Dynamics. 62, 1729–1763. DOI: https://doi.org/10.1007/s00382-023-06993-3
[41] Reboita, M.S., Ferreira, G.W.S., Ribeiro, J.G.M., et al., 2023. South American monsoon lifecycle projected by statistical downscaling with CMIP6-GCMs. Atmosphere. 14, 1380. DOI: https://doi.org/10.3390/atmos14091380
[42] Reboita, M.S., Ferreira, G.W.S., Ribeiro, J.G.M., et al., 2024. Assessment of precipitation and near-surface temperature simulation by CMIP6 models in South America. Environmental Research: Climate. 3(2), 025001. DOI: https://doi.org/10.1088/2752-5295/ad3fdb
[43] Dias, C.G., Reboita, M.S., 2021. Assessment of CMIP6 simulations over tropical South America. Revista Brasileira de Geografia Física. 14, 1282–1295. DOI: https://doi.org/10.26848/rbgf.v14.3.p1282-1295
[44] Lovato, T., Peano, D., 2020. CMCC CMCC-CM2-SR5 model output prepared for CMIP6 CMIP historical Version 20200616. Earth System Grid Federation. DOI: https://doi.org/10.22033/ESGF/CMIP6.3825
[45] Lovato, T., Peano, D., Butenschön, M., et al., 2022. CMIP6 simulations with the CMCC Earth System Model (CMCC-ESM2). Journal of Advances in Modeling Earth Systems. 14, e2021MS002814. DOI: https://doi.org/10.1029/2021MS002814
[46] Döscher, R., Acosta, M., Alessandri, A., et al., 2022. The EC-Earth3 Earth System Model for the climate model intercomparison project 6. Geoscience Model Development. 15, 2973–3020. DOI: https://doi.org/10.5194/gmd-15-2973-2022
[47] Krasting, J.P., John, J.G., Blanton, C.,et al., 2018. NOAA-GFDL GFDL-ESM4 model output prepared for CMIP6 CMIP historical. Version 20190726. Earth System Grid Federation. DOI: https://doi.org/10.22033/ESGF/CMIP6.8597
[48] Boucher, O., Denvil, S., Levavasseur, G., et al., 2018. IPSL IPSL-CM6A-LR model output prepared for CMIP6 CMIP historical. Version 20180803. Earth System Grid Federation. DOI: https://doi.org/10.22033/ESGF/CMIP6.5195
[49] Tatebe, H., Watanabe, M., 2018. MIROC MIROC6 model output prepared for CMIP6 CMIP historical. Version 20181212. Earth System Grid Federation. DOI: https://doi.org/10.22033/ESGF/CMIP6.5603
[50] Wieners, K.H., Giorgetta, M., Jungclaus, J., et al., 2019, MPI-M MPI-ESM1.2-LR model output prepared for CMIP6 CMIP historical. Version 20190710. Earth System Grid Federation. DOI: https://doi.org/10.22033/ESGF/CMIP6.6595
[51] Yukimoto, S., Koshiro, T., Kawai, H., et al., 2019. MRI MRI-ESM2.0 model output prepared for CMIP6 CMIP historical. Version 20190222. Earth System Grid Federation. DOI: https://doi.org/10.22033/ESGF/CMIP6.6842
[52] Hersbach, H., Bell, B., Berrisford, P., et al., 2020. The ERA5 global reanalysis. Quarterly Journal of Royal Meteorological Society. 146, 1999–2049. DOI: https://doi.org/10.1002/qj.3803
[53] Dullaart, J.C., Muis, S., Bloemendaal, N., et al., 2020. Advancing global storm surge modelling using the new ERA5 climate reanalysis. Climate Dynamics. 54, 1007–1021. DOI: https://doi.org/10.1007/s00382-019-05044-0
[54] Wilczak, J.M., Akish, E., Capotondi, A., et al., 2024. Evaluation and bias correction of the ERA5 reanalysis over the United States for wind and solar energy applications. Energies. 17, 1667. DOI: https://doi.org/10.3390/en17071667
[55] Karlsson, K.-G., Stengel, M., Meirink, J.F., et al., 2023. CLARA-A3: The third edition of the AVHRR-based CM SAF climate data record on clouds, radiation and surface albedo covering the period 1979 to 2023. Earth System Science Data. 15, 4901–4926. DOI: https://doi.org/10.5194/essd-15-4901-2023
[56] Boilley, A., Wald, L., 2015. Comparison between meteorological re-analyses from ERA-Interim and MERRA and measurements of daily solar irradiation at surface. Renewable Energy. 75, 135–143. DOI: https://doi.org/10.1016/j.renene.2014.09.042
[57] Lee, T., Singh, V.P., 2018. Statistical downscaling for hydrological and environmental applications, 1st edition. CRC Press: Florida, USA. pp. 181. DOI: https://doi.org/10.1201/9780429459580
[58] Tram-Anh, Q., Ngo-Duc, T., Espagne, E., et al., 2023. A 10-km CMIP6 downscaled dataset of temperature and precipitation for historical and future Vietnam climate. Scientific Data. 10, 257. DOI: https://doi.org/10.1038/s41597-023-02159-2
[59] Climate change 2021: The Physical Science Basis [Internet] [cited 2024 Mar 10]. Available from: https://www.ipcc.ch/report/ar6/wg1/#FullReport
[60] Logan, T., Aoun, A., Bourgault, P., et al., 2022. Ouranosinc/xclim: v0.37.0 (v0.37.0). Zenodo. DOI: https://doi.org/10.5281/zenodo.6671565
[61] Yilmaz, I.H., Mwesigye, A., 2018. Modeling, simulation and performance analysis of parabolic trough solar collectors: A comprehensive review. Applied Energy. 225, 135–174. DOI: https://doi.org/10.1016/j.apenergy.2018.05.014
[62] Bellos, E., Tzivanidis, C., Tsimpoukis, D., 2018. Enhancing the performance of parabolic trough collectors using nanofluids and turbulators. Renewable and Sustainable Energy Reviews. 91, 358–375. DOI: https://doi.org/10.1016/j.rser.2018.03.091
[63] He, Y., Yang, K., Wild, M., et al., 2023. Constrained future brightening of solar radiation and its implication for China's solar power. National Science Review. 10, nwac242. DOI: https://doi.org/10.1093/nsr/nwac242
[64] Solar Thermal Electricity: Global Outlook 2016—Full Report [Internet] [cited 2024 Mar 25]. Available from: https://www.estelasolar.org/wp-content/uploads/2016/02/GP-ESTELA-SolarPACES_Solar-Thermal-Electricity-Global-Outlook-2016_Full-report.pdf
[65] Pereira, E.B., Martins, F.R., Gonçalves, A.R., et al., 2017. Brazilian solar energy atlas, 2nd Edition. (In Portuguese). DOI: http://doi.org/10.34024/978851700089
[66] Renewable Energy Sources and Climate Change Mitigation—Special Report of the Intergovernmental Panel on Climate Change (IPCC) [Internet] [Accessed 2024 Mar 25]. Available from: https://www.ipcc.ch/report/renewable-energy-sources-and-climate-change-mitigation/
[67] Viviescas, C., Lima, L., Diuana, F.A., et al., 2019. Contribution of variable renewable energy to increase energy security in Latin America: Complementarity and climate change impacts on wind and solar resources. Renewable and Sustainable Energy Reviews. 113, 109232. DOI: https://doi.org/10.1016/j.rser.2019.06.039
[68] Li, J., Huo, R., Chen, H., et al., 2021. Comparative assessment and future prediction using CMIP6 and CMIP5 for annual precipitation and extreme precipitation simulation. Frontiers in Earth Science. 9, 2021. DOI: https://doi.org/10.3389/feart.2021.687976
[69] Anderson, T., Duke, M., Morrison, G., et al., 2009. Performance of a building integrated photovoltaic/thermal (BIPVT) solar collector. Solar Energy. 83, 445–455. DOI: https://doi.org/10.1016/j.solener.2008.08.013
[70] Trenberth, K.E., Fasullo, J., 2009. Global warming due to increasing absorbed solar radiation. Geophysical Research Letters. 36, L07706. DOI: https://doi.org/10.1029/2009GL037527
Downloads
How to Cite
Issue
Article Type
License
Copyright © 2024 Author(s)

This is an open access article under the Creative Commons Attribution-NonCommercial 4.0 International (CC BY-NC 4.0) License.