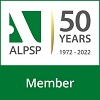
Uranium Species in Peat and Rock Sediments Near Sludge Storage Facility
DOI:
https://doi.org/10.30564/jees.v7i2.7831Abstract
The study aims to investigate uranium species in the sediments of the natural-technogenic system within a sludge storage facility in Russia. The relevance of this work is underscored by the need to assess the geochemical mobility of radionuclides, a critical factor for predicting their migration and environmental impact. The objective of the research was to determine the uranium species in both peat and sedimentary rock samples of the sludge storage facility and the adjacent area. Laboratory analyses included XRD, XRF analysis using synchrotron radiation, and scanning electron microscopy to study the composition and properties of minerals. The uranium species were further identified using a modified Tessier sequential extraction method. The results revealed that uranium predominantly occurs in a stable silicate-bound form (up to 80%) in sedimentary rocks, indicating minimal geochemical mobility. In contrast, in peat deposits, uranium is primarily associated with manganese and iron oxides (30–60%) as well as organic matter (5–40%), with the most mobile forms constituting less than 5%. The decrease in uranium concentration with distance from the facility was attributed to sorption onto organic matter and co-precipitation with mineral compounds, manganese and iron oxides, which serve as effective natural sorbents. The findings highlight the critical role of organic matter and metal oxides in limiting uranium migration, thus identifying them as key components in the formation of natural barriers for radionuclides. These results are crucial for assessing environmental risks associated with radioactive waste management and for developing strategies to minimize the ecological impact of sludge storages.
Keywords:
Uranium; Sludge Storage; Mineral Composition; Uranium Speciation in Sediments; Sorption by Peat; XRD; SEM; Safe ManagementReferences
[1] Letuvinkas A.I., 2002. Anthropogenic geochemical anomalies and the natural environment. NTL: Tomsk, Russia. pp. 1–92. (in Russian).
[2] Choppin, G.R., 2007. Actinide speciation in the environment. Journal of Radioanalytical and Nuclear Chemistry. 273, 695–703. DOI: https://doi.org/10.1007/s10967-007-0933-3
[3] Runde, W.H., 2000. The chemical interactions of actinides in the environment. Los Alamos Science. 26, 392–411
[4] Senko, J.M., Jonathan, D.I., Joseph, M.S., et al., 2002. In-situ evidence for uranium immobilization and remobilization. Environmental Science & Technology. 36(7), 1491–1496. DOI: https://doi.org/10.1021/es011240x
[5] Novikov, A.P., Vlasova, I.E., Safonov, A.V., et al., 2018. Speciation of actinides in groundwater samples collected near deep nuclear waste repositories. Journal of Environmental Radioactivity. 192, 334–341. DOI: https://doi.org/10.1016/j.jenvrad.2018.07.007
[6] Novikov, A.P., 2010. Migration and concentration of artificial radionuclides in environmental objects. Geochemistry International. 48, 1263–1387. DOI: https://doi.org/10.1134/S001670291013001X
[7] Istok, J.D., Senko, J.M., Krumholz, L.R., et al., 2004. Environmental Science & Technology. 38(2), 468–475. DOI: https://doi.org/10.1021/es034639p
[8] Romanchuk, A.Yu., Vlasova, I.E., Kalmykov, S.N., 2020. Speciation of uranium and plutonium from nuclear legacy sites to the environment: A mini review. Frontiers in Chemistry. 11(3), 61–65. DOI: https://doi.org/10.3389/fchem.2020.00630
[9] Majumder, E.L.-W., Wall, J.D., 2017. Uranium bio-transformations: Chemical or biological processes. Open Journal of Inorganic Chemistry. 7, 28–60. DOI: https://doi.org/10.4236/ojic.2017.72003
[10] Ure, A.M., Davidson, С.М., 2007. Chemical speciation in the environment, 2nd ed. Blackwell Science: Glasgow, Scotland. 1–452. DOI: https://doi.org/10.1002/9780470988312
[11] Newsome, L., Morris, K., Lloyd, J.R., 2014. The biogeochemistry and bioremediation of uranium and other priority radionuclides. Chemical Geology. 363, 164–184. DOI: https://doi.org/10.1016/j.chemgeo.2013.10.034
[12] Buddemeier, R.W., 1988. Transport of colloidal contaminants in groundwater: Radionuclide migration at the Nevada test site. Applied Geochemistry. 3, 535–548.
[13] Kersting, A.B., Efurd, D.V., Finnegan, D.L., et al., 1999. Migration of plutonium in ground water at the Nevada Test Site. Nature. 397(6714), 56–59
[14] McCarthy, J.F., 1989. Subsurface transport of contaminants. Environmental Science & Technology Journal. 23, 496–502
[15] Vilks, P., 1991. Sorption behaviour of 85Sr, 131I and 137Cs on colloids and suspended particles from the Grimsel test site, Switzerland. Applied Geochemistry. 6, 553–563
[16] Weisbrod, N., Dahan, O., Adar, E.M., 2002. Particle transport in unsaturated fractured chalk under arid conditions. Journal of Contaminant Hydrology. 56(2), 117–136. DOI: https://doi.org/10.1016/s0169-7722(01)00199-1
[17] Kalmykov, S., Zakharova, E., Novikov, A., et al., 2011. Effect of redox conditions on actinide speciation and partitioning with colloidal matter. In: Kalmykov, S., Denecke, M. (eds.). Actinide Nanoparticle Research. Springer: Berlin/Heidelberg, Germany. pp. 361–375. DOI: https://doi.org/10.1007/978-3-642-11432-8_13
[18] Wu, W.M., Carley, J., Luo, J., et al., 2007. In situ bioreduction of uranium (VI) to submicromolar levels and reoxidation by dissolved oxygen. Environmental Science & Technology Journal. 41(16), 5716–5723. DOI: https://doi.org/10.1021/es062657b
[19] Ilina, S.M., Marang, L., Lourino-Cabana, B., et al., 2020. Solid/liquid ratios of trace elements and radionuclides during a nuclear power plant liquid discharge in the seine river: Field measurements vs geochemical modeling. Journal of Environmental Radioactivity. 32560877, 220–221. DOI: https://doi.org/10.1016/j.jenvrad.2020.106317
[20] Shotyk, W., Bicalho, B., Cuss, C.W., et al., 2017. Trace metals in the dissolved fraction (<0.45μm) of the lower Athabasca River: Analytical challenges and environmental implications. Science of the Total Environment. 580, 660–669. DOI: https://doi.org/10.1016/j.scitotenv.2016.12.012
[21] Cuss, C.W., Grant-Weaver, I., Shotyk, W., 2017. AF4-ICPMS with the 300 da membrane to resolve metal-bearing “colloids” < 1kDa: Optimization, fractogram deconvolution, and advanced quality control. Analytical chemistry. 89(15), 8027–8035. DOI: https://doi.org/10.1021/acs.analchem.7b01427
[22] Di Bonito, M., Lofts, S., Groenenberg, J.E., 2018. Models of geochemical speciation: Structure and applications. In: De Vivo, B., Belkin, H.E., Lima, A. (eds.). Environmental Geochemistry: Site Characterization, Data Analysis and Case Histories, 2nd ed. Elsevier: Amsterdam, Netherlands. pp. 237–305. DOI: https://doi.org/10.1016/B978-0-444-63763-5.00012-4
[23] Krawczyk-Bärsch, E., Scheinost, A.C., Rossberg, A., et al., 2020. Uranium and neptunium retention mechanisms in Gallionella ferruginea/ferrihydrite systems for remediation purposes. Environmental Science and Pollution Research. 28, 18342–18353. DOI: https://doi.org/10.1007/s11356-020-09563-w
[24] Safonov, A.V., Perepelov, A.V., Babich, T.L., et al., 2020. Structure and gene cluster of the O-polysaccharide from Pseudomonas veronii A-6-5 and its uranium bonding. International Journal of Biological Macromolecules. 165, 2197–2204. DOI: https://doi.org/10.1016/j.ijbiomac.2020.10.038
[25] Rzhevskaia, A.V., Romanchuk, A.Y., Vlasova, I.E., et al., 2021. Partitioning of uranium in contaminated bottom sediments: The meaning of fractionation. Journal of Environmental Radioactivity. 1, 229–230. DOI: https://doi.org/10.1016/j.jenvrad.2021.106539
[26] Alekhin, Y.V., Ivleva, E.A., Il’ina, S.M., et al., 2020. Experimental fundamentals of the colloid hydrogeochemistry of continental runoff. Geochemistry International. 58(9), 1050–1060. DOI: https://doi.org/10.1134/S0016702920080030
[27] Winstanley, E.H., Morris, K., Abrahamsen-Mills, L.G., et al., 2019. U(VI) sorption during ferrihydrite formation: Underpinning radioactive effluent treatment. Journal of Hazardous Materials. 366, 98–104. DOI: https://doi.org/10.1016/j.jhazmat.2018.11.077
[28] Wiesmann, U., 1994. Biological nitrogen removal from wastewater. In: Ulber, R. (ed.). Biotechnics/Wastewater. Advances in Biochemical Engineering/Biotechnology. Springer: Berlin/Heidelberg, Germany. ABE(51), pp. 113–154.
[29] Toropov, A.S., 2020. Migration forms of anthropogenic radionuclides in tunnel waters at the Degelen mountains, Semipalatinsk test site. Geochemistry International. 58(3), 342–351. DOI: https://doi.org/10.1134/S0016702920020123
[30] Degueldre, C., Triay, I., Kim, J., et al., 2000. Groundwater colloid properties: A global approach. Applied Geochemistry. 15(7), 1043–1051. DOI: https://doi.org/10.1016/S0883-2927(99)00102-X
[31] De Jonge, M., Teuchies, J., Meire, P., et al., 2012. The impact of increased oxygen conditions on metal-contaminated sediments part I: Effects on redox status, sediment geochemistry and metal bioavailability. Water Research. 46(7), 2205–2214. DOI: https://doi.org/10.1016/j.watres.2012.01.052
[32] Malkovsky, V.I., Yudintsev, S.V., 2016. Model of colloidal transportation of radionuclides by groundwater. Doklady Earth Sciences. 470(1), 942–945. DOI: https://doi.org/10.1134/S1028334X16090051
[33] De Jonge, L.W, Moldrup, P., Rubæk, G.H., et al., 2004. Particle leaching and particle-facilitated transport of phosphorus at field scale. Vadose Zone Journal. 3(2), 462–470. DOI: https://doi.org/10.2136/vzj2004.0462
[34] Safonov, A., Lavrinovich, E., Emel’yanov, A., et al., 2022. Risk of colloidal and pseudo-colloidal transport of actinides in nitrate contaminated groundwater near a radioactive waste repository after bioremediation. Scientific Reports. 12(1), 15–21. DOI: https://doi.org/10.1038/s41598-022-08593-3
[35] Safonov, A.V., Boguslavskii, A.E., Boldyrev, K.A., et al., 2019. Biogenic factors of formation of geochemical uranium anomalies near the sludge storage of the Novosibirsk Chemical Concentrate Plant. Geochemistry International. 57, 709–715. DOI: https://doi.org/10.1134/S0016702919060090
[36] Boguslavsky, A., Shvartseva, O., Popova, N., et al., 2023. Biogeochemical in situ barriers in the aquifers near uranium sludge storages. Water. 15, 3020. DOI: https://doi.org/10.3390/w15173020
[37] Hilton, J., 1997. Aquatic radioecology post Chernobyl - a review of the past and a look to the future. Studies in Environmental Science. 68, 47–74
[38] Kumblad, L., Kautsky, U., Næslund, B., 2006. Transport and fate of radionuclides in aquatic environments – the use of ecosystem modelling for exposure assessments of nuclear facilities. Journal of Environmental Radioactivity. 87(1), 107–129. DOI: https://doi.org/10.1016/j.jenvrad.2005.11.001
[39] Choppin, G.R., Morgenstern A., 2001. Distribution and movement of environmental plutonium. Radioactivity in the Environment. 1, 91–105. DOI: https://doi.org/10.1016/S1569-4860(01)80009-7
[40] Hosseini, A., Thørring, H., Brown, J.E., et al., 2008. Transfer of radionuclides in aquatic ecosystems – default concentration ratios for aquatic biota in the Erica Tool. Journal of Environmental Radioactivity. 99(9), 1408–1429. DOI: https://doi.org/10.1016/j.jenvrad.2008.01.012
[41] Safonov, A.V., Boguslavsky, A.E., Boldyrev, K.A., et al., 2021. Geochemical modeling of the uranium behavior in groundwater near the sludge storages during bioremediation. Geochemistry International. 59(1), 56–65. DOI: https://doi.org/10.1134/S0016702921010080
[42] Boguslavsky, A.E., Gaskova, O.L., Naymushina, O.S., et al., 2020. Environmental monitoring of low-level radioactive waste disposal in electrochemical plant facilities in Zelenogorsk, Russia. Applied Geochemistry. 119, 104598. DOI: https://doi.org/10.1016/j.apgeochem.2020.104598
[43] Gaskova, O.L., Boguslavskii, A.E., Sirotenko, T.G., 2011. Geochemical composition of natural waters near a storage site of low-activity radioactive wastes. Water Resources. 38, 597–607. DOI: https://doi.org/10.1134/S0097807811050071
[44] Safonov, A.V., Boguslavsky, A.E., Gaskova, O.L., et al., 2021. Biogeochemical modelling of uranium immobilization and aquifer remediation strategies near NCCP sludge storage facilities. Applied Sciences. 11, 2875. DOI: https://doi.org/10.3390/app11062875
[45] Tessier, A., 1979. Sequential extraction procedure for the speciation of particulate trace metals. Analytical Chemistry. 51(7), 844–851.
[46] Odah, M.M., Naser, K.M., 2024. Effect of Humic acid and levels of Zinc and Boron on Chemical Behavior of Zinc in Calcareous Soil. Journal of Environmental & Earth Sciences. 6(3), 143–155. DOI: https://doi.org/10.30564/jees.v6i3.7126
[47] Taylor, S.R., 1964. Abundance of chemical elements in the continental crust: A new table. Geochimica et Cosmochimica Acta. 28(8), 1273–1285.
[48] Doynikova, O.A., Sidorenko, G.A., Mokhov, A.V., et al., 2015. New data on phosphosilicates of tetravalent uranium. New Data on Minerals. 50, 11–19. (in Russian).
Downloads
How to Cite
Issue
Article Type
License
Copyright © 2025 Olga Shvartseva, Daria Mashkova

This is an open access article under the Creative Commons Attribution-NonCommercial 4.0 International (CC BY-NC 4.0) License.