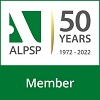
A Stable, Reliable, Cost-Effective Technique Route for Ni Detection in Industrial Wastewater via a Microfluidic Paper-Based Platform
DOI:
https://doi.org/10.30564/jees.v7i4.8433Abstract
Nickel (II) as one of the primary categories of heavy metals can lead to serious health problems if achieving the critical levels in the water. Thus, it is vital to propose a stable, reliable, and economical approach for detecting Ni ions. The microfluidic paper-based analytical devices (µPADs) are potential candidates for the detection of water quality parameters including pH, heavy ions, nitrite and so on. However, it suffers from a huge error caused by the environment and artificial mistakes. In this study, we proposed an improved technique route to increase the stability and reliability of microfluidic paper-based analytical devices. The main technique points include a stable light source, a matched camera, improved reliability of the devices, and effective calculated methods. Finally, we established 15 standard curves that could be used to detect nickel ions and obtained uniform colorimetric results with reliability and repeatability. With those improvements, the relative errors for the five types of real water samples from the Zhongshan industrial parks were reduced to 0.26%, 14.78%, 24.20%, 50.29% and 3.53%, respectively. These results were conducive to exploring this technique for the detection of nickel ions in wastewater from the Zhongshan industrial parks. The results demonstrated that the above technique route is promising for the detection of other heavy metal ions in industrial effluent.
Keywords:
Nickel Detection; Industrial Wastewater Detection; Microfluidic Paper-Based Chips; Analytical DeviceReferences
[1] Alabssawy, A.N., Hashem, A.H., 2024. Bioremediation of hazardous heavy metals by marine microorganisms: A recent review. Archives of Microbiology. 206(3), 103. DOI: https://doi.org/10.1007/s00203-023-03793-5
[2] Hashem, M.A., Hasan, M.A., Nayan, A.H., et al., 2021. The environmental impacts of heavy metals in soil, certain plants and wastewater near industrial area of Brahmanbaria, Bangladesh. Environmental Monitoring and Assessment. 193(10), 688. DOI: https://doi.org/10.1007/s10661-021-09497-x
[3] Lourembam, J., Haobam, B., Singh, K.B., et al., 2024. The molecular insights of cyanobacterial bioremediations of heavy metals: The current and the future challenges. Frontiers in Microbiology. 15, 1450992. DOI: https://doi.org/10.3389/fmicb.2024.1450992
[4] Jokić Govedarica, J., Tomašević Pilipović, D., Gvoić, V., et al., 2024. Eco-friendly nanoparticles: mechanisms and capacities for efficient removal of heavy metals and phosphate from water using definitive screening design approach. Environmental Geochemistry and Health. 46(4), 118. DOI: https://doi.org/10.1007/s10653-024-01879-7
[5] Karthik, V., Karuna, B., Kumar, P.S., et al., 2022. Development of lab-on-chip biosensor for the detection of toxic heavy metals: A review. Chemosphere. 299, 134427. DOI: https://doi.org/10.1016/j.chemosphere.2022.134427
[6] Mabowa, H.M., Mkhohlakali, A., Mokoena, S., et al., 2024. Removal of nickel from nickel sulfite-fire assay dissolution filtrate through precipitation. ACS Omega. 9(5), 5592–5600. DOI: https://doi.org/10.1021/acsomega.3c07623
[7] Kumar, A., Kumar, V., Thakur, M., et al., 2023. Comprehensive review of nickel biogeochemistry, bioavailability, and health risks in the environment. Land Degradation & Development. 34(14), 4141–4156. DOI: https://doi.org/10.1002/ldr.4775
[8] Ramadan, F., Nour, H.E., Wahed, N.A., et al., 2024. Heavy metal contamination and environmental risk assessment: a case study of surface water in the Bahr Mouse stream, East Nile Delta, Egypt. Environmental Monitoring and Assessment. 196(5), 429. DOI: https://doi.org/10.1007/s10661-024-12541-1
[9] Serafin, J., Kishibayev, K., Tokpayev, R., et al., 2023. Functional activated biocarbons based on biomass waste for CO2 capture and heavy metal sorption. ACS Omega. 8(50), 48191–48210. DOI: https://doi.org/10.1021/acsomega.3c07120
[10] Manwani, S., Bhoot, N., Pandey, H., et al., 2022. Mitigation of lead and Cadmium from vegetable crops through different biochemical adsorbent treatments at Sanganer industrial area, Jaipur. Materials Today: Proceedings. 69, 1556–1564. DOI: https://doi.org/10.1016/j.matpr.2022.07.059
[11] Barik, D., Anilkumar, A., Porel, M., 2024. Solid-state fluorescent organic polymers for visual detection and elimination of heavy metals in water. ACS Polymers Au. 4(5), 428–437. DOI: https://doi.org/10.1021/acspolymersau.4c00048
[12] Fakayode, S.O., Walgama, C., Fernand Narcisse, V.E., et al., 2023. Electrochemical and colorimetric nanosensors for detection of heavy metal ions: A review. Sensors. 23(22), 9080.
[13] Handa, S., Singh, P., Prakash, B., et al., 2024. Evaluation of heavy metal contamination: An analogy between conventional techniques and paper microfluidics as the futuristic probe for diverse environmental matrices. Bulletin of Materials Science. 47(4), 262. DOI: https://doi.org/10.1007/s12034-024-03292-3
[14] Li, Y., Peng, G., He, Q., et al., 2015. Dispersive liquid–liquid microextraction based on the solidification of floating organic drop followed by ICP-MS for the simultaneous determination of heavy metals in wastewaters. Spectrochimica Acta Part A: Molecular and Biomolecular Spectroscopy. 140, 156–161. DOI: https://doi.org/10.1016/j.saa.2014.12.091
[15] Fendrych, K., Porada, R., Baś, B., 2023. Electrochemical sensing platform based on Zeolite/Graphite/Dimethylglyoxime nanocomposite for highly selective and ultrasensitive determination of nickel. Journal of Hazardous Materials. 448, 130953. DOI: https://doi.org/10.1016/j.jhazmat.2023.130953
[16] Nadumane, S.S., Biswas, R., Mazumder, N., 2024. Integrated microfluidic platforms for heavy metal sensing: A comprehensive review. Analytical Methods. 16(18), 2810–2823. DOI: https://doi.org/10.1039/d4ay00293h
[17] Martinez, A.W., Phillips, S.T., Butte, M.J., et al., 2007. Patterned paper as a platform for inexpensive, low-volume, portable bioassays. Angewandte Chemie. International Edition in English. 46(8), 1318–1320. DOI: https://doi.org/10.1002/anie.200603817
[18] Zhao, X., Ding, Z., Chen, H., et al., 2023. Acoustofluidics-assisted multifunctional paper-based analytical devices. Analytical Chemistry. 96(1), 496–504. DOI: https://doi.org/10.1021/acs.analchem.3c04603
[19] Martínez-Pérez-Cejuela, H., Calabretta, M.M., Michelini, E., 2024. Chemiluminescence “add-and-measure” Sensing paper based on the prussian blue/metal–organic framework MIL-101 nanozyme for rapid hydrogen peroxide detection. Analytical Chemistry. 96(42), 16561–16569. DOI: https://doi.org/10.1021/acs.analchem.4c02340
[20] Aryal, P., Boes, J., Brack, E., et al., 2024. Fill, fold, photo: Preconcentration and multiplex detection of trace level heavy metals in water. ACS Sensors. 9(10), 5479–5488. DOI: https://doi.org/10.1021/acssensors.4c01708
[21] Mazur, F., Tjandra, A.D., Zhou, Y., et al., 2023. Paper-based sensors for bacteria detection. Nature Reviews Bioengineering. 1(3), 180–192. DOI: https://doi.org/10.1038/s44222-023-00024-w
[22] Zhao, X., He, Y., Shao, S., et al., 2024. CRISPR/Cas14 and G-quadruplex DNAzyme-driven biosensor for paper-based colorimetric detection of african swine fever virus. ACS Sensors. 9(5), 2413–2420. DOI: https://doi.org/10.1021/acssensors.4c00090
[23] Ashaiba, A., Sapna, K., Arun, A.B., et al., 2024. Development and evaluation of a noninvasive microfluidic-based paper analytical device for leptospirosis diagnosis. Analytical Chemistry. 96(29), 11997–12005. DOI: https://doi.org/10.1021/acs.analchem.4c01934
[24] Li, Y., Guo, Y., Chen, H., et al., 2024. Flexible wearable plasmonic paper-based microfluidics with expandable channel and adjustable flow rate for portable surface-enhanced raman scattering sweat sensing. ACS Photonics. 11(2), 613–625. DOI: https://doi.org/10.1021/acsphotonics.3c01490
[25] Steijlen, A.S.M., Parrilla, M., Van Echelpoel, R., et al., 2023. Dual microfluidic sensor system for enriched electrochemical profiling and identification of illicit drugs on-site. Analytical Chemistry. 96(1), 590–598. DOI: https://doi.org/10.1021/acs.analchem.3c05039
[26] Campu, A., Muresan, I., Craciun, A.-M., et al., 2023. Innovative, flexible, and miniaturized microfluidic paper-based plasmonic chip for efficient near-infrared metal enhanced fluorescence biosensing and imaging. ACS Applied Materials & Interfaces. 15(48), 55925–55937. DOI: https://doi.org/10.1021/acsami.3c08658
[27] Wu, H., Chen, J., Lin, P., et al., 2024. Nanozyme-catalyzed colorimetric detection of the total antioxidant capacity in body fluids by paper-based microfluidic chips. ACS Applied Materials & Interfaces. 16(30), 39857–39866. DOI: https://doi.org/10.1021/acsami.4c07835
[28] Singh, S., Raucci, A., Cimmino, W., et al., 2024. Paper-based analytical devices for cancer liquid biopsy. Analytical Chemistry. 96(9), 3698–3706. DOI: https://doi.org/10.1021/acs.analchem.3c04478
[29] Xu, B., Ding, Y., 2022. Hydrophilic/hydrophobic SiO2 nanoparticles enabled janus‐type paper through commercial glaco spraying and air‐plasma treatment. Advanced Materials Interfaces. 9(21), 2200934. DOI: https://doi.org/10.1002/admi.202200934
[30] Cao, Y., Sun, Y., Yu, R.-J., et al., 2023. Paper-based substrates for surface-enhanced Raman spectroscopy sensing. Microchimica Acta. 191(1), 8. DOI: https://doi.org/10.1007/s00604-023-06086-2
[31] Romanholo, P.V.V., de Andrade, L.M., Silva-Neto, H.A., et al., 2024. Digitally controlled printing of bioink barriers for paper-based analytical devices: An environmentally friendly one-step approach. Analytical Chemistry. 96(14), 5349–5356. DOI: https://doi.org/10.1021/acs.analchem.3c03801
[32] Filippidou, M.-K., Chatzandroulis, S., 2023. Microfluidic devices for heavy metal ions detection: A review. Micromachines. 14(8), 1520. DOI: https://doi.org/10.3390/mi14081520
[33] Kulkarni, R.M., Sunil, D., 2024. Small molecule optical probes for detection of H2S in water samples: A review. ACS Omega. 9(13), 14672–14691. DOI: https://doi.org/10.1021/acsomega.3c08573
[34] Zhang, Y., Yu, Y., Yang, X., et al., 2024. Pb(II) inhibits CRISPR/Cas12a activation and application for paper-based microfluidic biosensor assisted by smartphone. Sensors and Actuators B: Chemical. 398, 134732. DOI: https://doi.org/10.1016/j.snb.2023.134732
[35] Fan, K., Zeng, J., Yang, C., et al., 2022. Digital quantification method for sensitive point-of-care detection of salivary uric acid using smartphone-assisted μPADs. ACS Sensors. 7(7), 2049–2057. DOI: https://doi.org/10.1021/acssensors.2c00854
[36] Yang, R., Cheng, W., Chen, X., et al., 2018. Color space transformation-based smartphone algorithm for colorimetric urinalysis. ACS Omega. 3(9), 12141–12146. DOI: https://doi.org/10.1021/acsomega.8b01270
[37] Lin, Y., Ye, S., Tian, J., et al., 2023. Paper-assisted ratiometric fluorescent sensors for on-site sensing of sulfide based on the target-induced inner filter effect. Journal of Hazardous Materials. 459, 132201. DOI: https://doi.org/10.1016/j.jhazmat.2023.132201
[38] Chaiyo, S., Kunpatee, K., Kalcher, S., et al., 2024. 3D Paper-Based Device Integrated with a Battery-Less NFC Potentiostat for Nonenzymatic Detection of Cholesterol. ACS Measurement Science Au. 4(4), 432–441. DOI: https://doi.org/10.1021/acsmeasuresciau.4c00012
[39] Xiong, X., Guo, C., Yan, G., et al., 2022. Simultaneous Cross-type Detection of Water Quality Indexes via a Smartphone-App Integrated Microfluidic Paper-Based Platform. ACS Omega. 7(48), 44338–44345. DOI: https://doi.org/10.1021/acsomega.2c05938
Downloads
How to Cite
Issue
Article Type
License
Copyright © 2025 Xiuxia Li, Qing’er Yao, Jiangyue Bai, Zihang Wang, Xiaolu Xiong, Zifan Ning, Songhe Liu, Shiqi Xu, Chunpan Zhang, Yujiu Jiang, Mingxu Chu, Yanbo Yang, Dong Jiang, Junfeng Han

This is an open access article under the Creative Commons Attribution-NonCommercial 4.0 International (CC BY-NC 4.0) License.